Impact areas
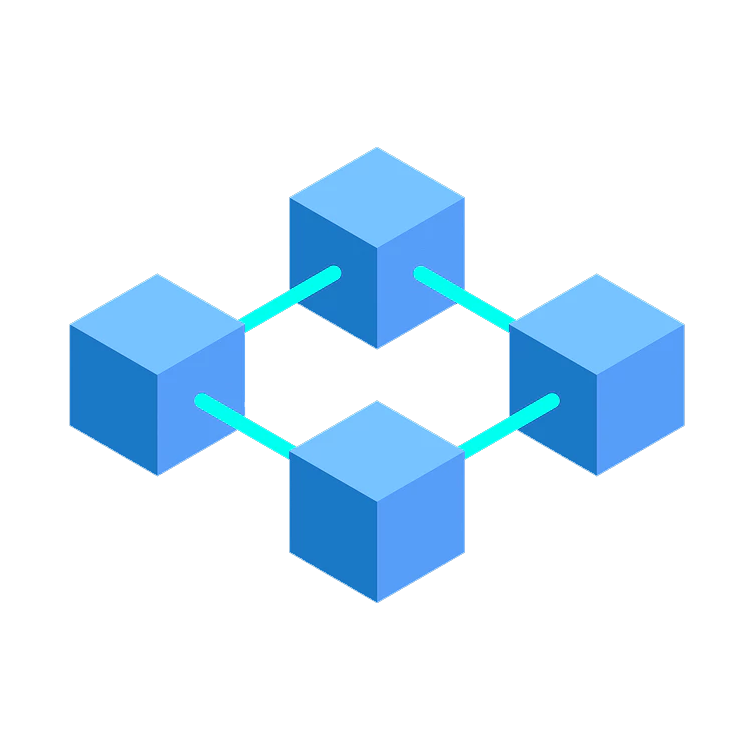
Generations of sTES technologies
As advancements in seasonal Thermal Energy Storage (TES) have progressed through generations, from the pioneering pilot projects to the current fourth-generation focus on effective storage integration, numerous improvements have been made. New waterproofing and thermal insulation materials have already been applied in the second generation. Efficiencies were thus increased (e.g. through optimised loading and unloading systems) and the first problems (especially leakages) were solved at the same time. In the third generation, the priority shifted to cost reductions, for instance by using prefabricated elements for thermal insulations or structural elements of TTES. The new insulation techniques especially bottom thermal insulation improved the efficiencies, while composite foils with vapour diffusion layers and testable waterproofing techniques further reduced water losses. Today, at the fourth-generation level, most attention focuses on effective storage integration and operation in larger networks. This is complemented by tuning temperature levels and combining different energy sources. Nevertheless, several unresolved critical issues remain that require further attention. Issues are attributed to the next, fifth generation. Technically, improvements and new developments of suitable materials are needed. Achieving long-term robustness is a widespread challenge of existing sites, e.g. due to structural fatigue of waterproofings. TES need to keep energy losses at a minimum over a lifetime of several decades, not only for the sake of storage efficiency but also to minimise environmental risks. However, in practice, suitable monitoring and control systems are required to save the ambient ground and groundwater environment. Regarding TES operation, the vast opportunities to integrate new and diverse energy sources are still not exploited. Most TES rely on solar energy, but smart integration in heating and cooling networks may also facilitate industrial excess energy, geothermal energy, and waste heat from office buildings and data centres. Aside from this, the optimal use of TES requires attuned control engineering.
Finally, a future approach for more flexibility is the consideration of combined storage systems that represent multi-storage solutions of different sizes and different temperature levels. Such solutions offer not only more flexibility but also can be upgraded more easily in case of network expansion or innovative materials. Such modular implementation of TES can also reduce the high initial costs of construction, which are often decisive for the realisation of a project.

Low-ex(ergy) (LowEx) grids
LowEx, short for Low Exergy, refers to systems or grids that aim to minimize the use of exergy in various processes. Exergy is a measure of the useful energy within a system that is available to do work. It takes into account both the quantity and quality of energy.
LowEx grids focus on reducing the overall exergy consumption in energy systems, with an emphasis on efficiency and sustainability. These grids are designed to optimize the use of energy resources by minimizing waste and losses in the conversion and distribution processes. The goal is to enhance energy efficiency, reduce environmental impact, and promote the use of renewable and low-impact energy sources.
In the INTERSTORES, the LowEx grid and the whole energy system (sources, sinks, hydraulics, operational facilities) are characterised, and a monitoring concept of the energy system for automatic data recording and access is designed. For environmental and economic analyses, previous and projected relevant data are collected (e.g., material and energy consumption) and all other site-specific information is compiled in the INTERSTORES database.
INTERSTORES aims to provide customised solutions for different use cases, including both heating and cooling (in expanded LowEx network) applications. It offers novel, multi-functional, and modular sTES solutions, which also convert excess power into heat using power-to-heat technologies, promoting grid stability and reducing costs.
Environmental relevance
Environmental effects, impacts and benefits of the sTES will be assessed within a life cycle assessment (LCA) framework. This evaluation method traces the entire flow of energy and the use of resources necessary for a product or service. In such a “cradle-to-grave” approach, all up- and downstream inputs and outputs along all the phases of the life cycle of an sTES are analysed and evaluated.
LCA follows the ISO 14040-14049 series of standards and will be elaborated complying with the International Reference Life Cycle Data System of the EC.
Special focus will be on the development of an LCA framework that is component-based, and which can consider quantitatively the recycling of components, incl. materials and services. INTERSTORES will also expand existing LCIA by novel impact evaluation concepts (e.g., based on distance-to-target principle). This will be developed based on simulated thermal environmental effects and build up on own previous work on ecological assessment and resource use.
As a result, both site-specific as well as general environmental benefits of the storage solutions will be elaborated (contrasting single-sTES variants and systems without sTES), and environmentally optimised field implementations will be identified.
The application of the developed LCA framework will reveal environmental performances of the sTES demos, and of sTES variants in general (based on generalised scenario analyses), and it will be used to formulate a user-friendly streamlined LCA framework that can be coupled in a multi-criteria assessment framework and used by third parties.
Integrity of structural materials
Supporting measures such as the shotcreting of cavern walls and manufactured structural elements will be adapted to the specific conditions of a CTES. Some designs pose a challenging environment for long-term material durability (e.g., our Giga-CTES demo VECTES). Such a facility will create an operating environment for cementitious binder-based materials (CBBM) with hydrothermal exposure, hydrostatic pressure, cyclic temperature, water within the CTES and ambient groundwater, which represents a unique knowledge gap for these materials.
The integrity of CBBM and any other structural materials is essential to ensure the operability and fulfilment of long service life (100 years) while guaranteeing economic viability. So far published literature refers to the effects of the elevated temperature on dry CBBM, which are extensively studied in conjunction with fire exposure. However, limited insight exists regarding saturated concrete and mid-range elevated temperatures such as tackled by the Giga-CTES of INTERSTORES Project.
System integration
The performance of an sTES is governed by engineered realisation, and it strongly depends on the energy system integration. While there exists a considerable knowledge of heating/cooling systems, operation and simulation, embedding of optimised sTES is a delicate task.
Advancing the market readiness of any novel sTES variant requires a systemic model representation that comprises storage components, its environment, and the connected system. Our goal is to realise and contrast two completely different sTES concepts, fundamental differences in optimal system integration of Reno- sTES and Giga-CTES need to be considered.
In the INTERSTORES Project, three timespans for flexibility can be considered: short-term storage application, seasonal operation and long-term, multi-annual system evolution/expansion. Especially for short-term flexibility and failure analysis, smart monitoring concepts that resolve particular thermal conditions in sTES and ambient ground are needed. This is realised ideally as a digital twin that resembles the conditions of ambient ground, storage and energy system. In the most advanced form, the digital twin also integrates machine learning techniques that are trained by process-based models or learn from historical data and forecast events. INTERSTORES will open a new door and develop a digital process twin, for virtual representation, control and optimisation of the sTES variants.
Storage performance
The criteria for optimal storage performance are technical, economic and environmental. By system representation and integrated planning based on the digital twin, holistic techno-economic assessments can not only guide system optimal planning and control, but they are crucial to demonstrate the market competitiveness of sTES. Both sTES variants and the integrating energy systems will be reproduced as digital process twins which are developed in synchronised working steps at both sites and maintained in chief responsibility by AIT. They will be modular and this concept is crucial to capture the compartment-specific processes on appropriate time and space resolution and to enable computationally efficient model updating (data assimilation, inversion, calibration). Finally, the modular concept will ease parallel development and efficient collaboration during the Project.
Market potential
To enhance the replication of sustainable energy storage (sTES) technologies with significant market potential, INSTERSORES will follow various strategies. This includes transforming digital simulation and control tools into user-friendly INTERSTORES software for effective planning, optimization, control, and prediction across different Reno-sTES and Giga-CTES sites. Also, a comprehensive guideline will be developed, incorporating recommendations for site-specific monitoring requirements and a SWOT analysis. The replication process will involve exploring sTES replication and refining software applicability through theoretical implementations at selected transfer sites. Identifying key features of high-potential sites and conducting a generalized assessment of regional market potentials, including considerations for large-scale integration, will be crucial. Additionally, INSTERSORES aims to create a roadmap for technology development by leveraging emerging sTES technologies expected to reach maturity in the next 5-10 years.
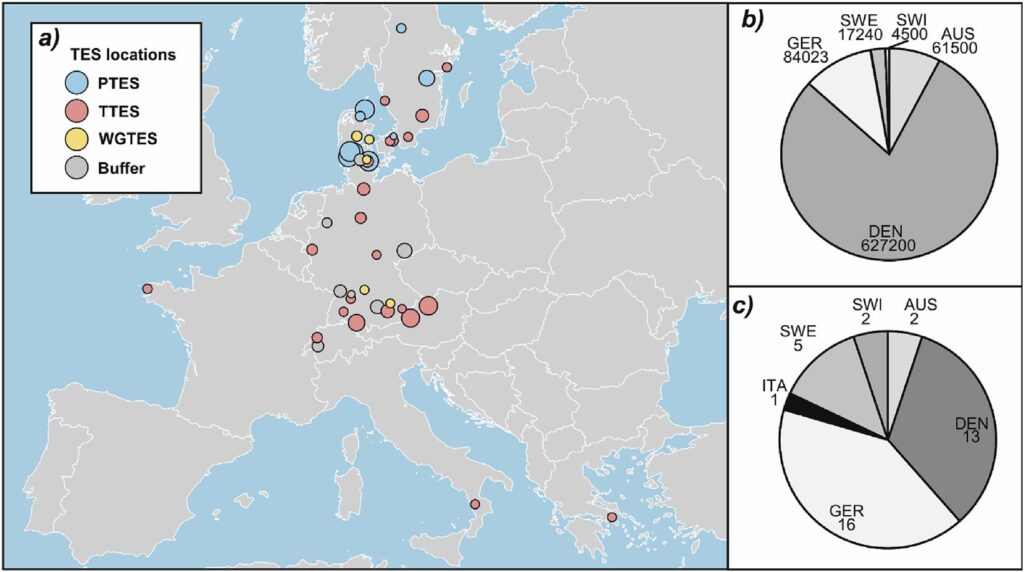